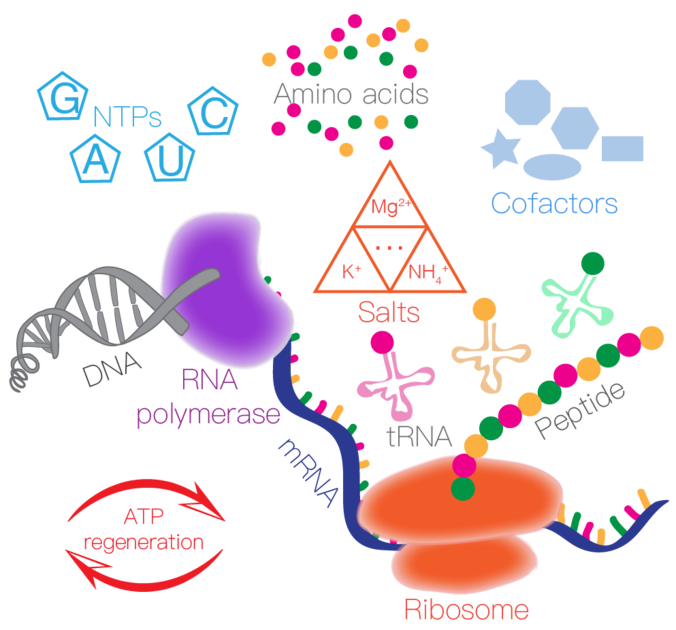
Classic and emerging areas
Did and doing
1. Development of versatile cell-free systems
For fundamental and applied research.
Based on the direct control of transcription and translation, two main types of cell-free systems have been constructed and developed. One is an extract-based system using different prokaryotic and eukaryotic cells; the other is a reconstituted system (PURE system). Cell-free systems have been used for decades as a tool in fundamental and applied research, and now they are being transformed into factories with commercial feasibility for making products.
2. (Protein synthesis) × N
Cell-free system was born for making proteins from genes.
The advantage, compared to biosynthesis in living cells, is that cell-free biosynthesis is a faster way to obtain an expressed phenotype (protein) from a genotype (gene) in a few hours. The lack of cell growth means that biological reactions in cell-free systems can be directly controlled in the open environment. Therefore, proteins such as membrane proteins, complex proteins and toxic proteins that are hard to be made in living cells can be readily prepared in cell-free systems.
3. PTMs
For accurate post-translational modifications (PTMs).
Protein post-translational modification (PTM) increases the functional diversity of the proteins. These modifications include glycosylation, phosphorylation, ubiquitination, nitrosylation, methylation, acetylation, lipidation and proteolysis and influence almost all aspects of normal cell biology and pathogenesis. Future regulation of pharmaceutical proteins may change toward requirements of a more uniform protein production and controlled PTM process. Use of cell lysates of various cell based systems already applied for glycoengineering, which could be either native- or metabolically engineered-cell lines. Prokaryote or eukaryote-based PTM cell-free protein synthesis systems are being developed, which help to avoid PTM heterogeneity.
4. Expanding the genetic code
Highly-efficient incorporation of unnatural amino acids (UNAAs).
The ability to biologically incorporate UNAAs into proteins opens a whole new world that enables the expansion of our ability to customize completely new proteins with novel functions never encountered in nature. In vitro cell-free systems have been used as the testing platform to make it work. Moreover, in biomanufacturing, in vitro cell-free systems also have great advantages over in vivo cell systems for UNAA incorporation, including broad suitability and applicability, high efficiency, easy use of UNAAs, no cell membrane barrier problem, high toxicity tolerance, and easy multiple UNAA incorporation.
5. Prototyping
Rapid prototyping of synthetic biological circuits.
In the post-genomic era, cell-free systems have the potential to become one of the most important technologies for functional genomics and proteomics. The engineering of biological systems can be readily carried out in a cell-free context. Cell-free systems can be directly used for various applications. Alternatively, initial deployment of synthetic systems in a cell-free context can help to fast track the development of living cell synthetic systems.
6. Synthetic metabolic engineering
Simple technology for designing a chimeric metabolic pathway.
Speeding up the design-build-test (DBT) cycle is a fundamental challenge facing bioengineering. Cell-free metabolic engineering will facilitate efforts to define, manipulate, and understand metabolic pathways for accelerated DBT cycle without the need to reengineer organisms. With this technique, functional aspects of complex protein machines – such as how combinations of different enzymes interact, and how close together they must be to perform coupled reactions – can be tested directly.
7. Biological materials
Genetically encoded self-assembly.
The field of biological materials is an emerging discipline at the intersection of molecular biology, chemistry, physics, and materials engineering. Multidisciplinary cell-free synthetic biology has been developed to make genetically encoded self-assembled biomaterials. It involves directly mimicking biological processes to produce materials with enhanced or unusual properties. Some biomaterials such as virus-like particles and DNA-protein hybrid materials have been successfully fabricated.
8. Artificial cell
For better and smarter cell.
An artificial cell is an engineered capsule in which bioactive materials are encapsulated within a membrane to perform designated functions. Its construction can help to understand the origin of life, and its applications range from the environment to the healthcare. Three basic elements are needed for the bottom-up construction of a living artificial cell to perform the essential functions of life. These include cell membrane, information-carrying molecule DNA or RNA, and metabolism system. In this context, bottom-up cell-free synthetic biology is becoming a powerful transcription/translation toolbox to engineer artificial cells. The greatest potential applications of artificial cells lie in the biomedical therapeutics.
9. High-throughput screening
Finding novel things on demand and quickly.
Cell-free systems can serve as a test bed to quickly characterize biological parts and can act as a high-throughput screening system for drug discovery. Starting with linear DNA templates, assays can be finished in a few hours. Cell-free systems can greatly facilitate the future development of biopharmaceuticals and biocatalysts. High-throughput workflow typically is operated using plate readers, flow cytometry, or microfluidics. Their open nature means that cell-free systems can be easily interfaced with nonbiological devices, such as microbeads, which enable instant analysis in a high-throughput workflow.
10. Biosensoring
New-generation portable biosensors
Diagnosing the disease from a patient’s body fluid is considered as the most expected way in modern diagnostics. Synthetic biology provides tools to sense biomolecular targets and show the signal information. However, the major biosensing applications focus on the living cells, with the panic of biosafety. The development of cell-free synthetic biology is suitably overcoming this challenge because of no cell growth. Compared to whole cell biosensor, cell-free systems receive a wider range of sensing options, not only chemical molecular but also nucleic acids (RNA, DNA). For this concept, cell-free synthetic biology has been employed for infectious disease detection.
Copyright reserved by Lu Group@Tsinghua University
Lu Group website: www.lugroup.net; Email: yuanlu@tsinghua.edu.cn
We keep updating continuously.